
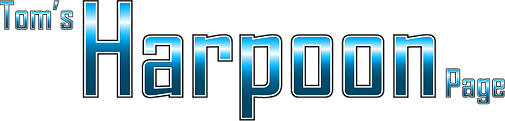
Surface-to-Air
Arleigh Burke IIA vs. YJ-18A
In this example we will explore Arleigh Burke IIA defensive capability against sea-skimming anti-ship missiles (or Anti-Ship Cruise Missiles - ASCM) - the main menace modern ship faces.
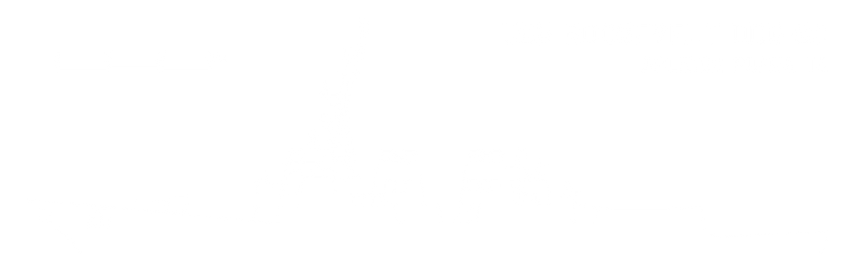
Let's say USS Roosevelt (DDG-80) is sailing through South China Sea, on a sunny day in a nice calm weather. Suddenly she is attacked by a volley of YJ-18A - Chinese knock off of Russian 3M054 Klub. Instead of fixed number of missiles attacking the ship, we will assume volley to be infinite in order to check how many missiles Chinese need to launch in order to have a reasonable chance of getting at least one through.
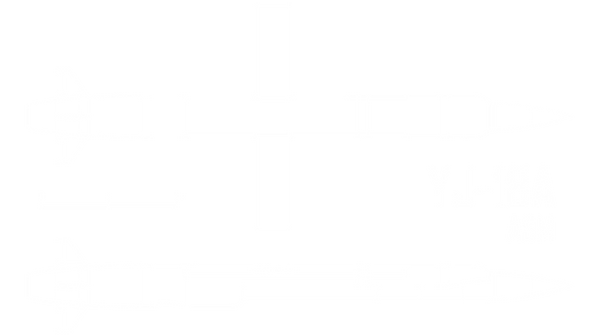
YJ-18A is a sea-skimming missile, which means it travel toward its target as close to the waves as possible (at a cruising speed of 528 kts), in order to avoid detection (more on that later). Its design is peculiar in that, when it acquires its target and gets within 11 nm of it, it launches the terminal vehicle, which is a small rocket, accelerated to mach 3.0. It supposes to skip through the last line of defenses and make it harder to hit.
Area Defense
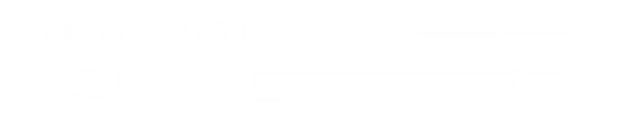
Roosevelt's first line of defense against air threats are its surface-to-air missiles. Although it has few types of them, RIM-162 ESSM (Evolved Sea Sparrow) is the missile that is best suited for the situation, so we will focus on it.
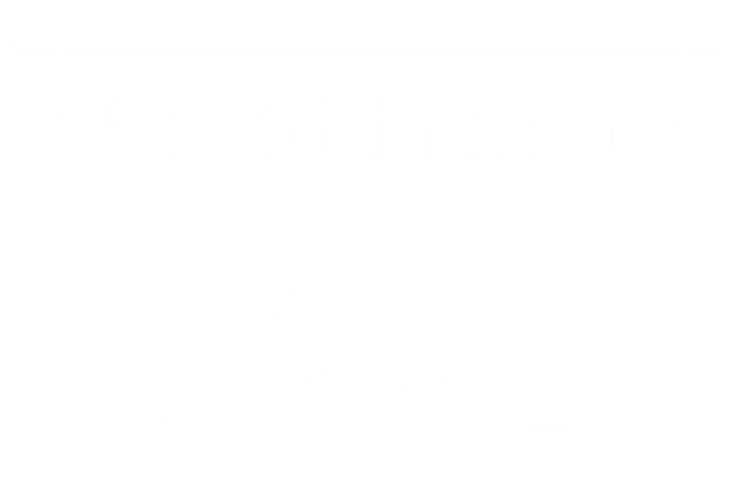
Arleigh Burke contains 32 ESSMs in three VLS (Vertical Launch System) mounts. VLS (as far as mounts goes) can fire all around the ship in 360 degree coverage. This will be somewhat limited by fire control radars, but we'll get to that.
For this part of the example, we will use Surface-to-Air Engagement flowchart. I advice gettingt it and follow through with it handy.

In order to intercept ASCMs, they need to be detected first. Low flying objects, like ASCMs, can be detected by Air Search (AS), Low-Altitude Search (LAS) and 3D (3D - duh) radars. They can actually be detected by surface search radars too, but at so reduced range that they are useless in defense. Roosevelt's main air detection system is SPY-1D radar - probably the most sophisticated, integrated air search system in the world. It utilizes four big phased array antennas to search and track air contacts (this is not knowledge necessary to play the game, but let's learn something and immerse ourselves in the topic).
How far away the target can be detected depends on a few factors. First one is the base radar range. It depends on radar power, shape of antenna, processors and whole lot of other things that we do not need to be concerned with. In game it boils down to just one number, which is the range in nautical miles (nm) that target will be detected at. This ranges, for all radars, are listed in Annex J1.
​
For SPY-1D they look like this:

As you can see, how far the target can be detected, also depends on the target's radar signature. This is how much radar energy is reflected by the target. Larger targets reflect more energy and thus can be detected farther away than small targets (although with modern stealth technology this is not always the case).
YJ-18A's radar signature is VSmall, so the range at which SPY-1D can detect it is 63 nm.
We write this value into AS, HF or 3D Radar box on the flowchart.
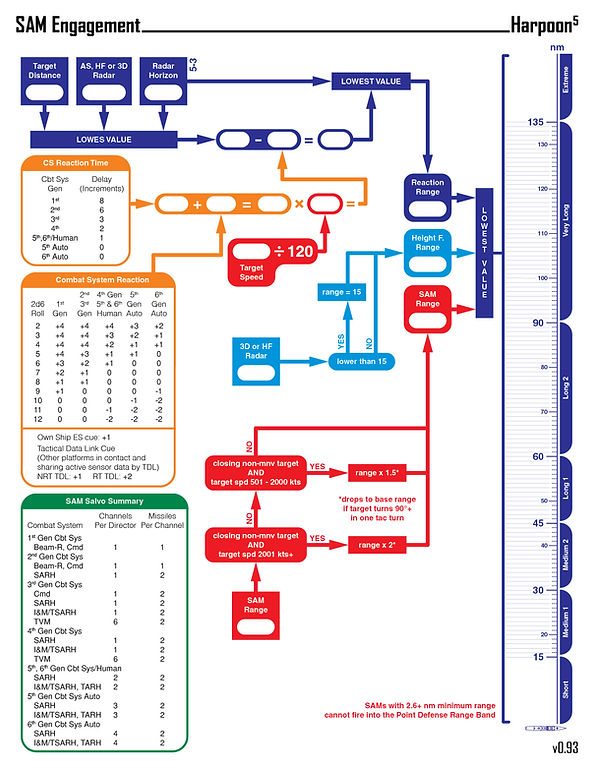
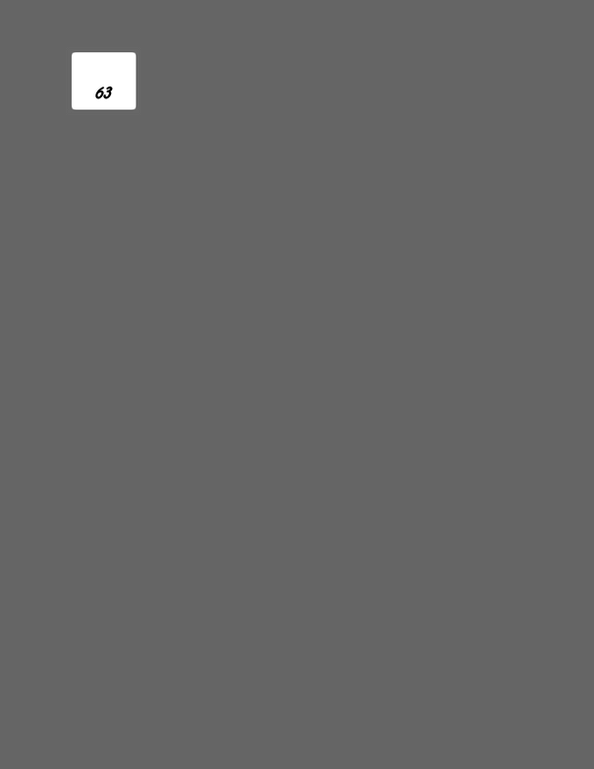
For sea-skimming targets, clutter effect from sea waves can be a serious problem, that affects this range value. But in good weather that we assumed, and with SPY-1D's clutter resistance, we can skip this part for now and discuss it in a different example.
It doesn't matter from which direction missiles come - shipborne search radars have 360 degree coverage.
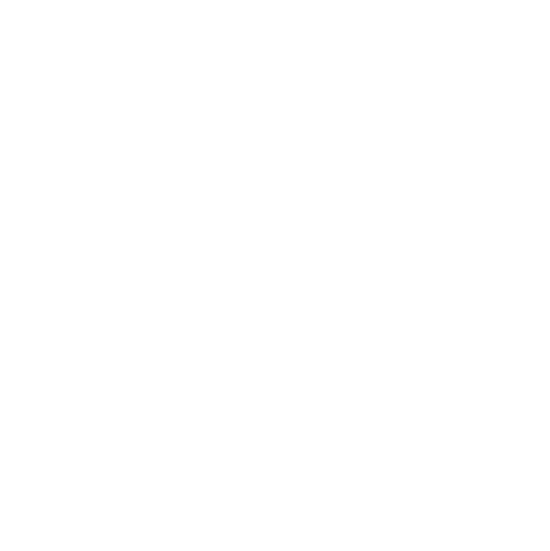
This 63 nm is the farthest away the SPY-1D can detect very small (VSmall) air target in the best of conditions (well, in one situation it can actually detect it farther, but it will be mentioned later). But conditions are not always perfect, and many factors will reduce your detection range. The biggest of them is what is called radar horizon.

Because Earth is round (regardless of what some people say), and radar waves don't bend over its curvature (normally at least, yeah nothing is simple), how far radar can detect targets depends on radar's and target's height above ground (or water in our case). Higher the detecting radar and/or target, farther detection is possible. This is the reason, why modern anti-ship missiles fly as low as they can, because it exposes them to radar detection closer to target and leave less time for reaction.
​
To check the effects of radar horizon, we go to Radar Line of Sight table on page 5-3 of the rulebook.
​
Here we cross observing unit's height with target's height. For shipborne radars we use ship's size from its Annex data. Arleigh Burke's size is Medium.
Don't confuse size with signature. Although both of them are usually the same, in Burke's case the signature is smaller than size, as she is built with incorporation of stealth techniques.
​
Now we find the height of YJ-18A's flight under VLow column.
​
Crossing both of them we find that radar horizon from medium ship to very low flying target is limited to 24 nm.
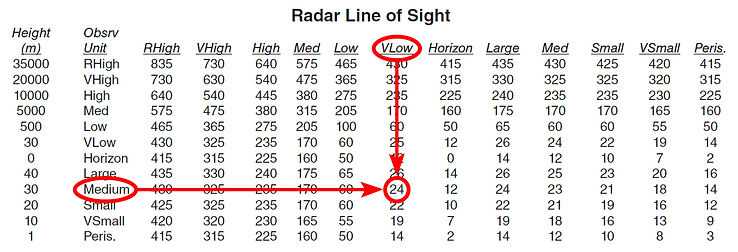
We write this value into the Radar Horizon box on the flowchart.
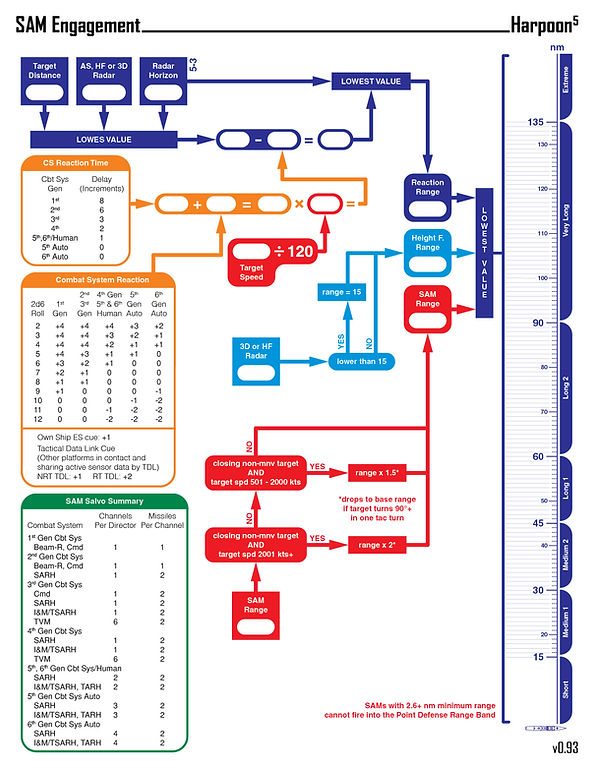
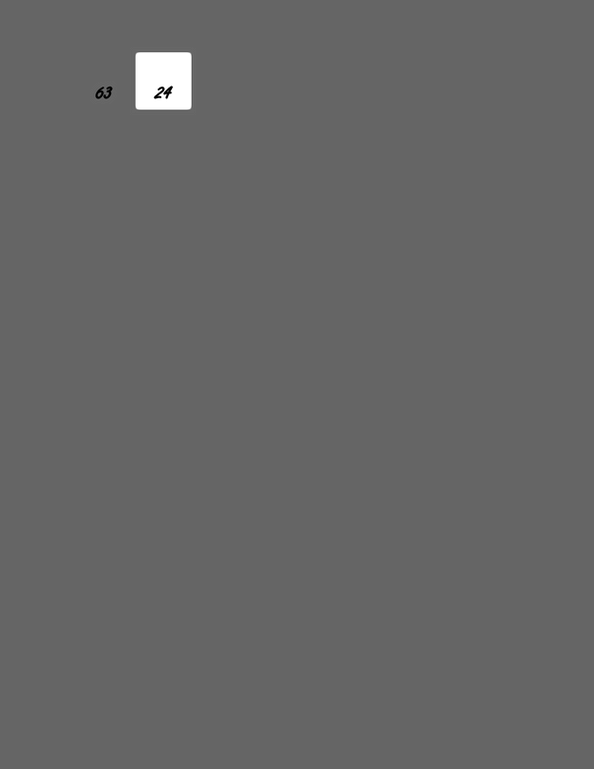
Now we add the distance at which missiles are launched. It can be important if they are launched inside a reaction envelope, by let's say a submarine. But since we assume the worst case scenario for the Chinese, we will place the launch point somewhere outside the radar horizon, let's say 60 nm out. We write it into the Target Distance box on the flowchart.
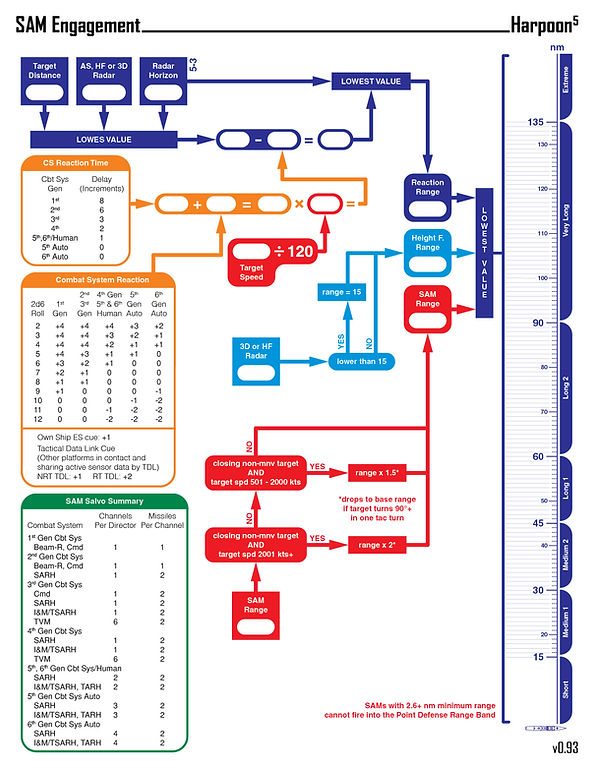
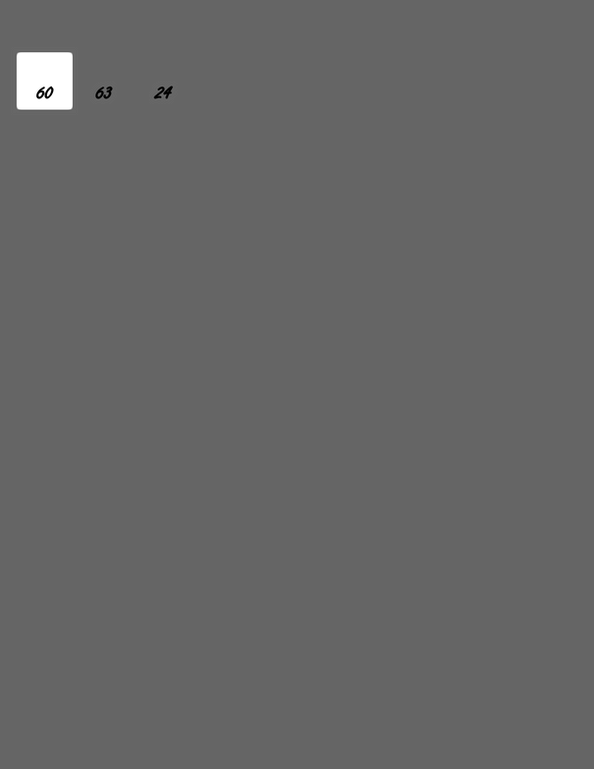
From this three values we take the lowest one - in our case radar horizon value of 24 nm - and transfer it following the arrow. This will be the basis to calculate the distance at which the ship reacts to the threat.
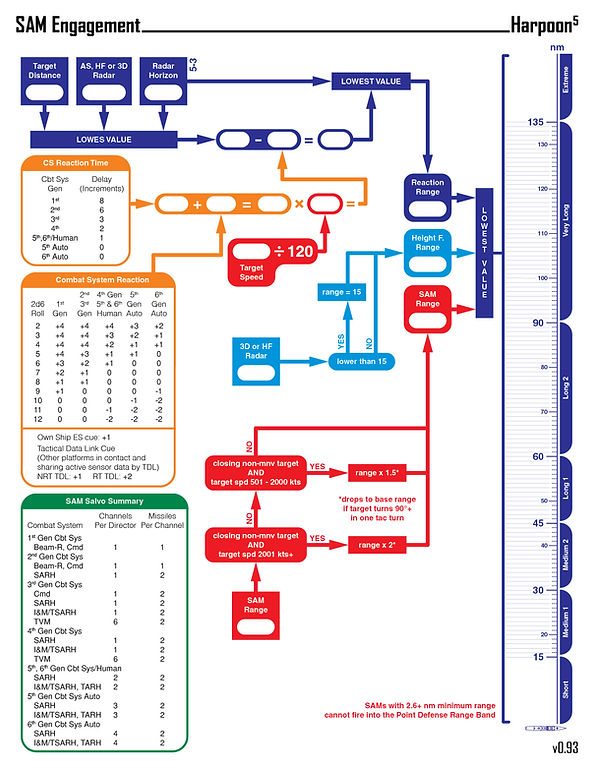

Now we check combat system reaction time. The fact that a threat came into range does not mean it is automatically found and recognized. Identifying a target and aiming weapons at it takes time. It takes more time if the fire control system is old and has slow computing power or needs much of the process to be done manually by humans in the loop. In extreme cases, the system might not react in time to fire, before ASCMs reaches the ship.
​
First we check base combat system reaction time, based on the system's generation, found in the ship's data annex. An Arleigh Burke IIA is equipped with 6th generation automated combat system, the best there is so far, so its reaction time is very good. Checking the CS Reaction Time table, Gen 6th Auto system has a base reaction time of 0.
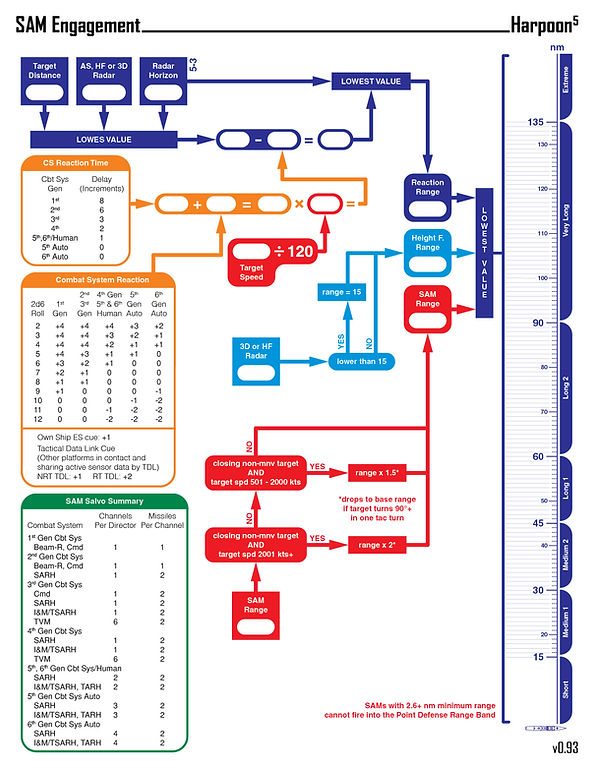
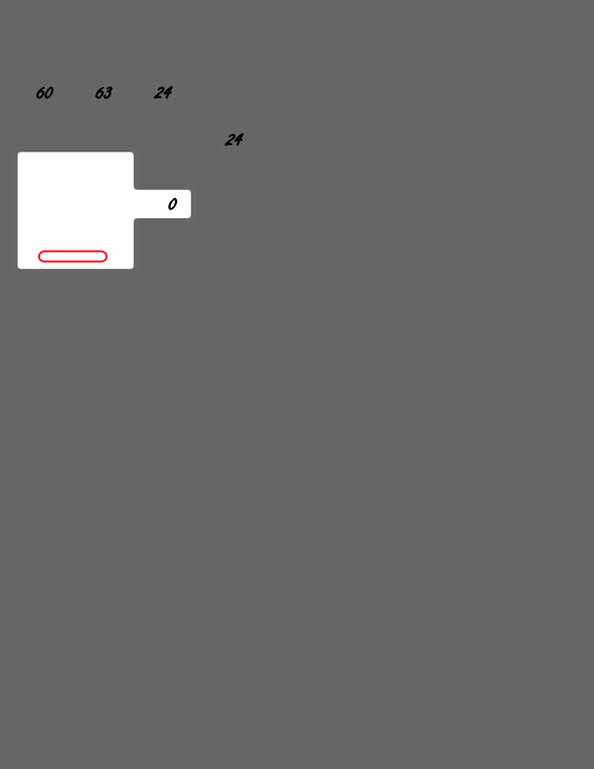
This reaction time can be further modified by various events. Maybe the radar beam was in the opposite direction from the target when it got in range, or the radar operator spilled coffee on the screen or got sleepy, or any number of factors.
​
To check this additional delay we roll 2D6 and check the rolled sum against system's generation on Combat System Reaction table (there can be modifiers to this roll, but none are used in this situation). 6th Gen Auto system has modifier span from +2 to -2. Negative modifiers lower reaction time and improve ship's situation.
​
Because we consider the worst case scenario for Chinese, we assume Roosevelt rolling whooping 11 on 2D6, getting -2 modifier to the reaction time. We write it down in proper box and sum it with base reaction time. In our case it is 0 plus -2 for the total of -2.
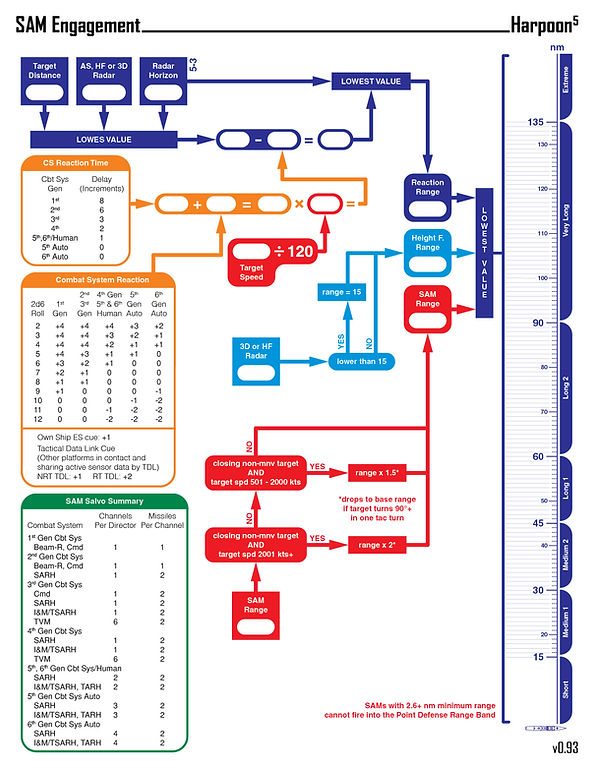
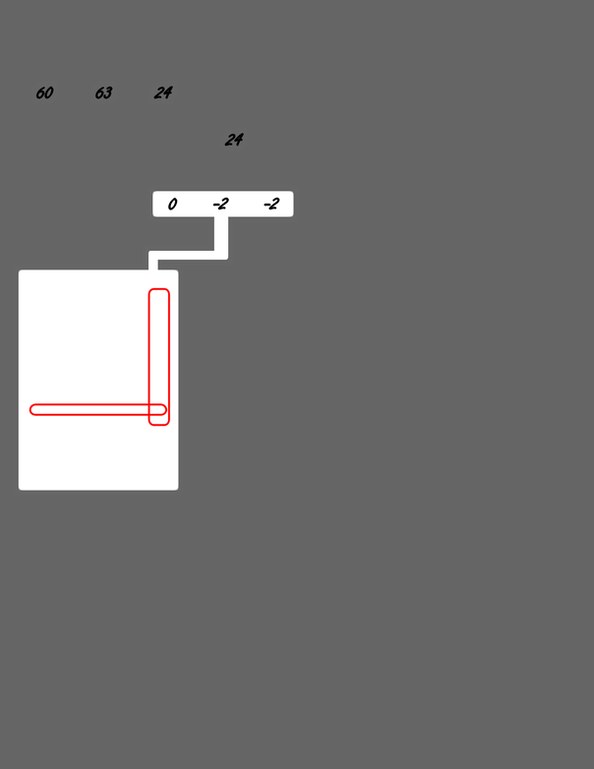
Now we will use the delay to modify the reaction range. Delay is measured in a number of so called increments. A basic Tactical Turn of Harpoon represents 3 minutes of real time, but it can be divided into six 30 seconds increments for fast moving action. This increments are now used to calculate final reaction range.
​
Every increment of delay means 30 seconds of ASCMs flying inside the defenses' envelope without being engaged. So how close a ship is able to engage incoming ASCMs depends not only on duration of delay but also speed of the cruise missiles. The faster the missile, the more ground it covers in each increment.
​
Speed of the YJ-18A is 528 kts. We write it into Target Speed box. Next we divide it by 120 and write the outcome into the box following the arrow - in our case it is 4.4. This 4.4 is the number of nautical miles YJ-18A travels each 30 second increment. So we multiply this distance by the number of delay increments and write it next to the reaction base range, following the arrow - in our case -8.8.
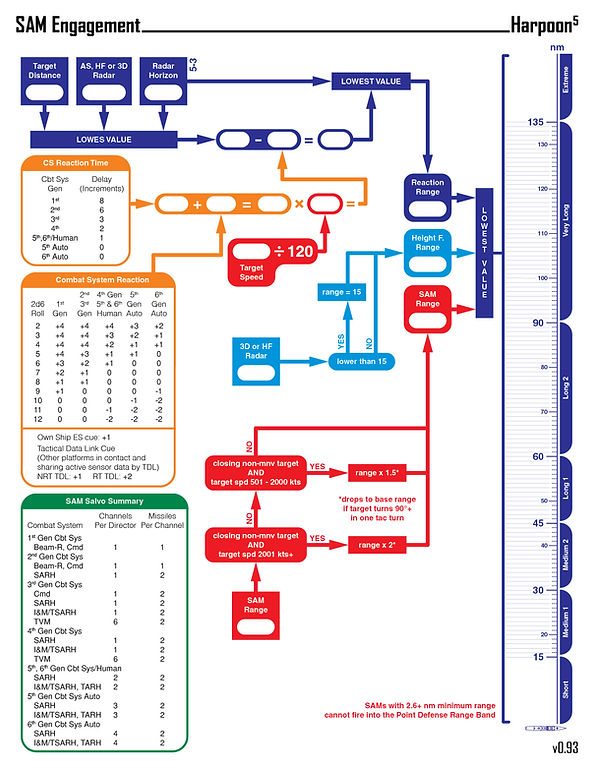
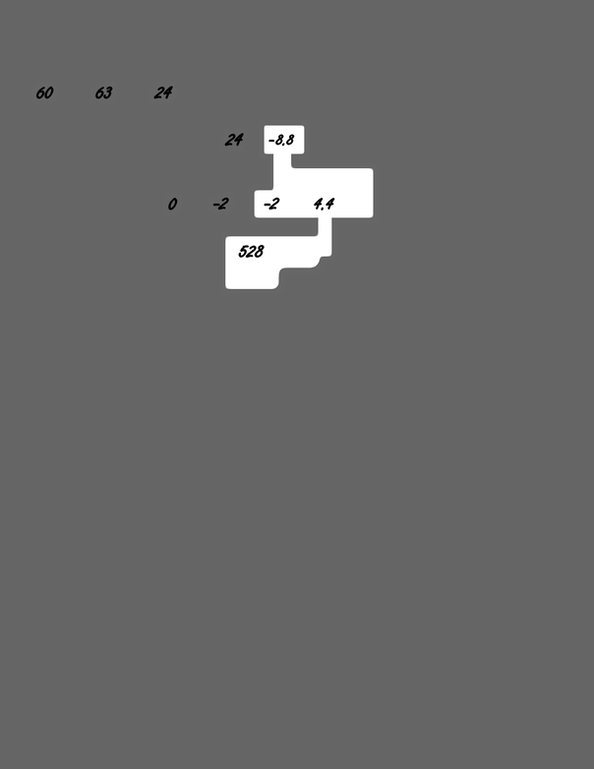
Most of the time you will subtract the value from the previous step from the base reaction range, making the reaction late, but in our special case, we will add this value, extending the reaction range from 24 to 32.8 nm.
​
Although this early reaction can extend radar's base range, at which detection is made, it still cannot go over the horizon. So we make final comparison of this range to the radar horizon range.
​
Since it's now higher than our radar horizon, we use the radar horizon range again and write it as final Reaction Range.
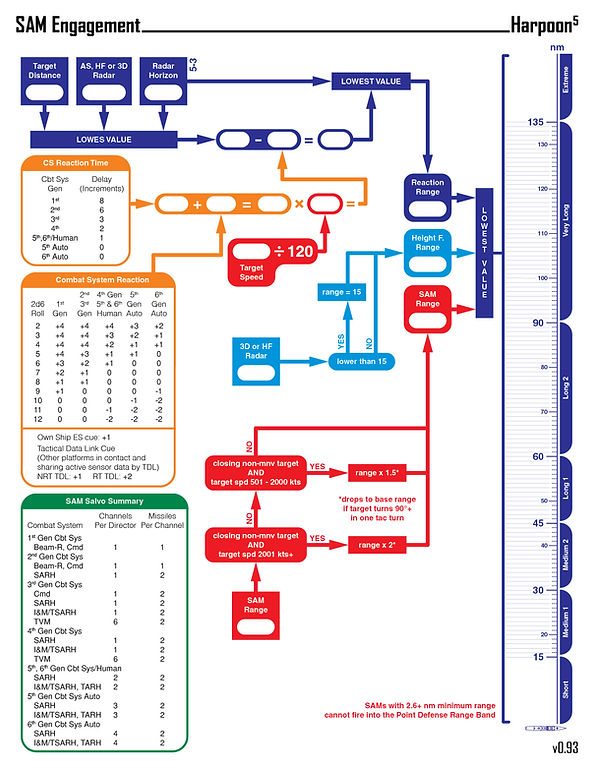

To accurately shoot at flying targets beyond 15 nm, combat system need to have the target's altitude data provided. This is done by dedicated height finding radars (HF), or by 3D radars which provide both bearing and altitude.
​
Since SPY-1D is the latter type it is used for both detection and height finding. We can thus copy range of 63 nm from AS, HF or 3D Radar box into 3D or HF Radar box.
​
Then, since targets closer than 15 nm don't need altitude data to be provided, we check if radar's range is shorter than 15 nm, and if it is, we write 15 into Height F. Range box.
​
Since SPY-1D's range is much higher than 15 nm, we transfer it into Height R. Range box.
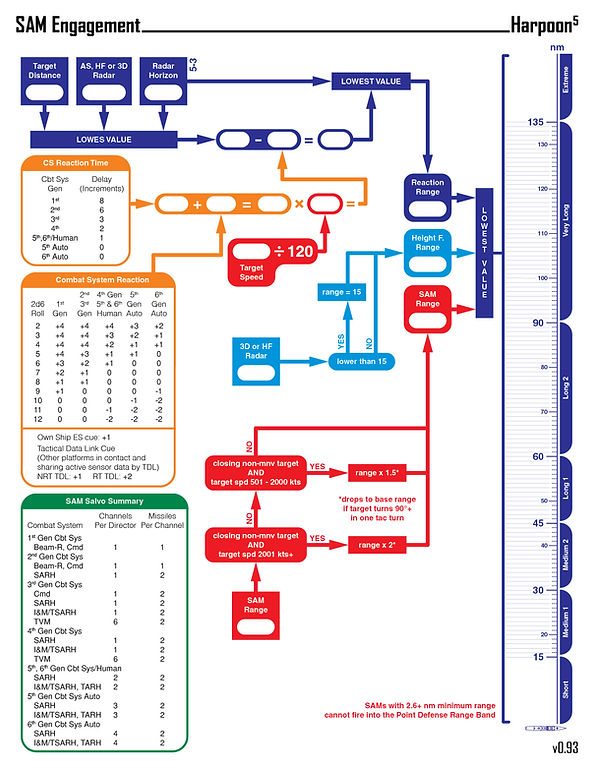
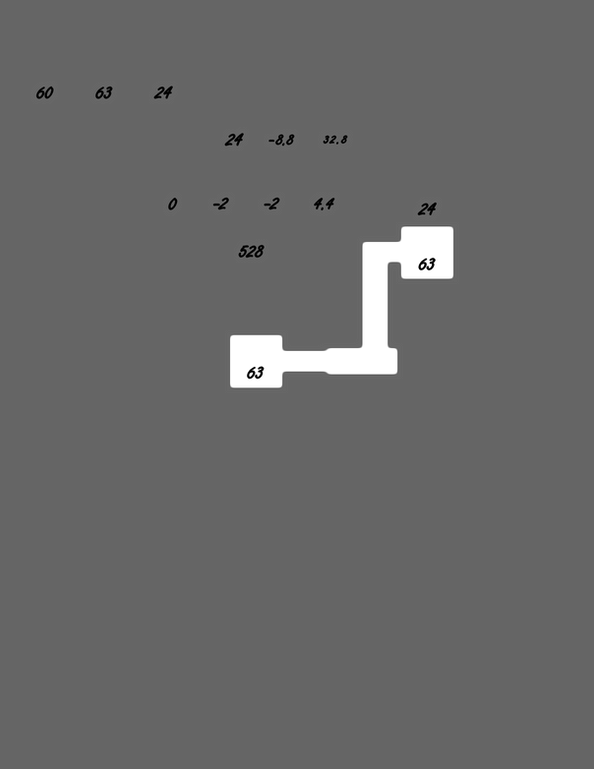
We already have two limiting factors to engagement range - detection and height finding. Now we check the last one - weapon range. Though air tagets can be detected even hundreds of nautical miles from the ship, weapons to engage them might not have sufficient range to get there.
​
ESSM's range, taken from the data annex, is 27 nm. It is a base range that we put into SAM Range box.
​
This is base range against maneuvering targets. When the target is trying to escape the attack, the missile pursuing it loses energy. If missile's target is not trying to evade, the missile has more energy that can be used to reach farther and intercept target at a longer distance.
​
Also, if a non-maneuvering target is very fast, defending missiles can be launched when it is farther away.
​
Moving along the arrow we check if the attacking target's speed is 2001 kts or more. It is not, but it is between 501 and 2000 kts (528 kts), so we multiply the base range of 27 nm times 1.5 getting 40.5 nm range, at which ESSM can reach YJ-18A.
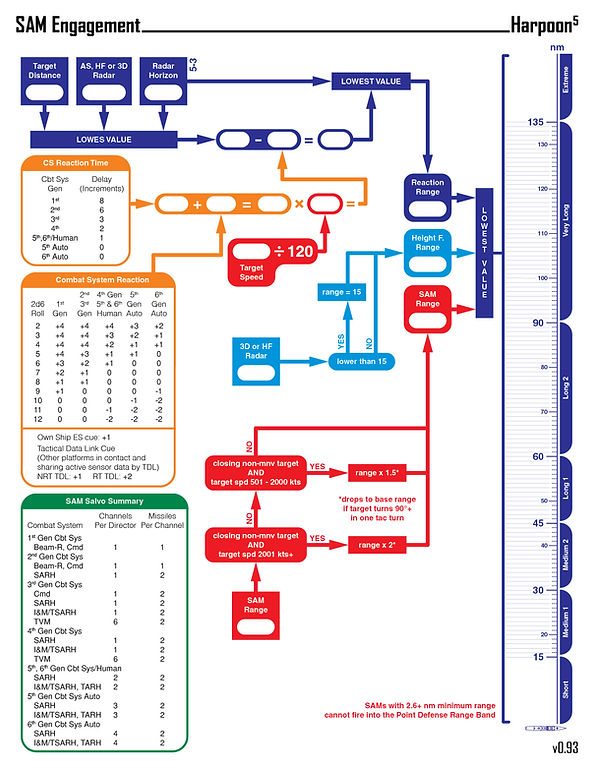
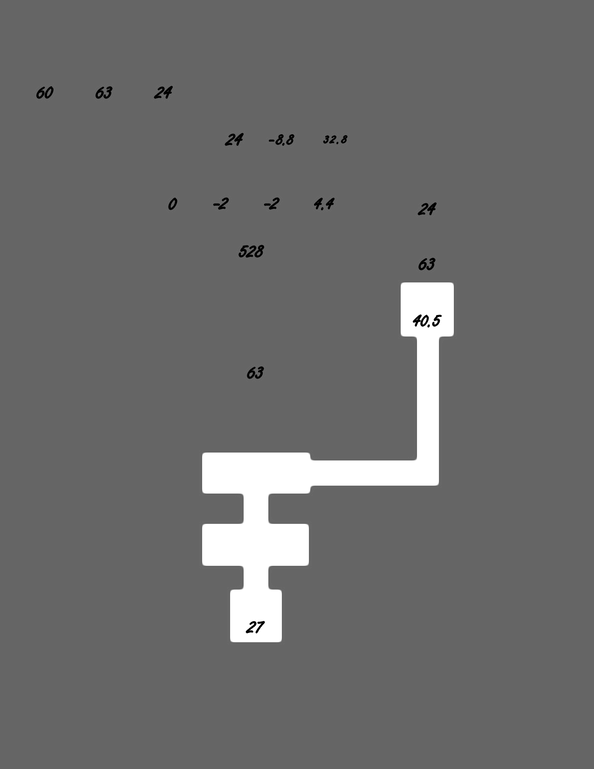
Having these three values we find which one of them is the lowest - in our example it's 24 nm. We mark it on the ruler on the right of the flowchart. It shows us in what range bracket interception takes place. In our example it's Medium 1.
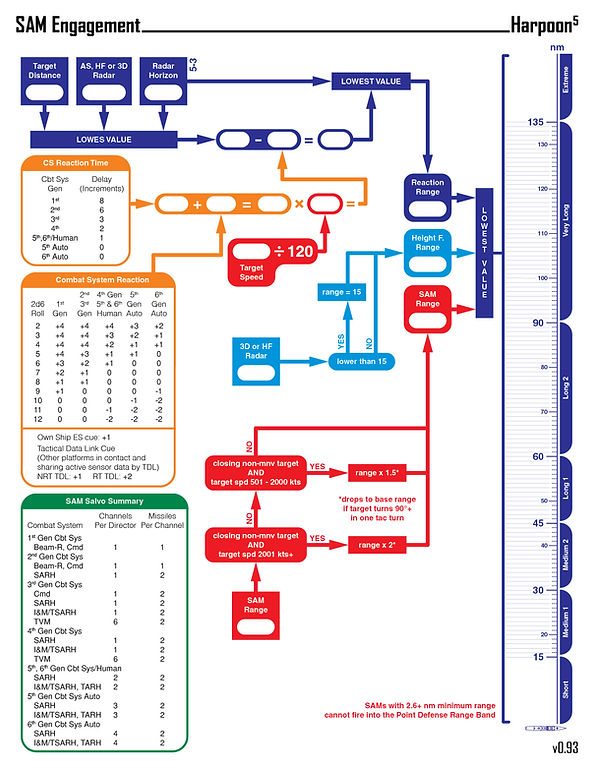
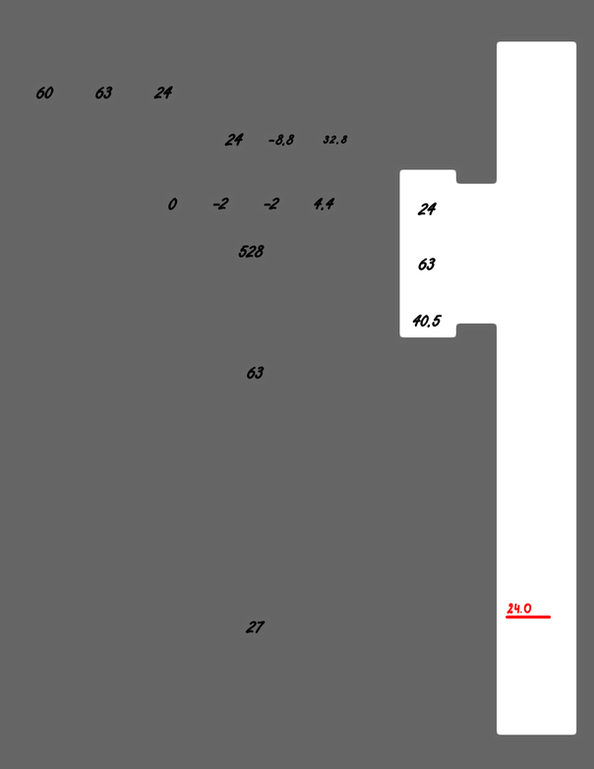
Knowing intercept range, now we check the number of engagements the system can make against incoming threats. Number of engagements are listed in the intercept tables, which are in the rulebook on pages from 8-5 to 8-7.
​
First we find the right table by looking up one that is for the altitude at which interception takes place. Ours is SAM Intercept Table - VLow Altitude Targets.
​
Then we find sub-table, by finding proper interception range bracket - Medium 1 in our case.
​
Next we check target's speed to find proper column. Speed of sound at Low and VLow altitude is 660 kts. Since YJ-18A's speed is below that (528 kts) it is Subsonic target, so we use first column.
​
The row we use is of course 6th Auto, given our combat system generation.
​
This gives us M-3S-P or one engagement in medium bracket, three in short bracket and one in point defense bracket.

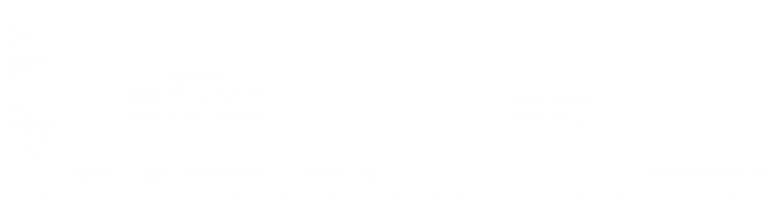
Because YJ-18A is a very peculiar cruise missile, we need to modify the number of engagements for one thing. YJ-18A has what is called "sprint vehicle". At the last leg, before hitting the target, the missile detaches its warhead and accelerate it to mach 3 during the last 10.8 nm of flight.
​
This reduces short bracket engagements by one. So the final number of engagements is M-2S-P.
Each engagement does not mean one attack. Number of attacks per engagement depends again on combat system's generation and number of weapon directors.
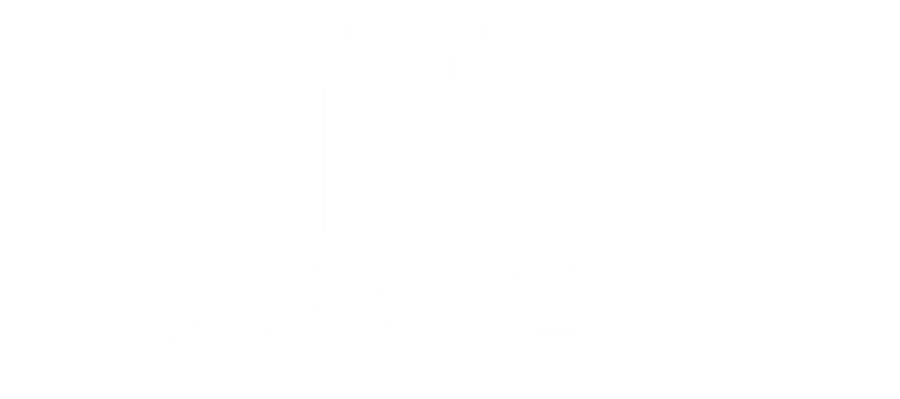
Weapon directors are special radars that guide missiles to their targets. Arleigh Burke posses three SPG-62 radars for missile guidance - one fore and two aft.

ESSM's guidance is of Semi-Active Radar Homing (SARH) type, which means that director is sending radar signals that are bounced of the target and received by ESSM's receiver. The ESSM is following this bounced signals toward the target.

Looking at SAM Salvo Summary table we see that the 6th Generation Auto system has 4 Channels Per Director and 2 Missiles Per Channel. This means that each director can target up to four different threats and guide up to two missiles at each of them simultaneously. It can also dedicate more than one channel to the same target. For instance it can attack two targets with four missiles each at the same time, although given the deadliness of ESSMs, that would be a waste of missiles.
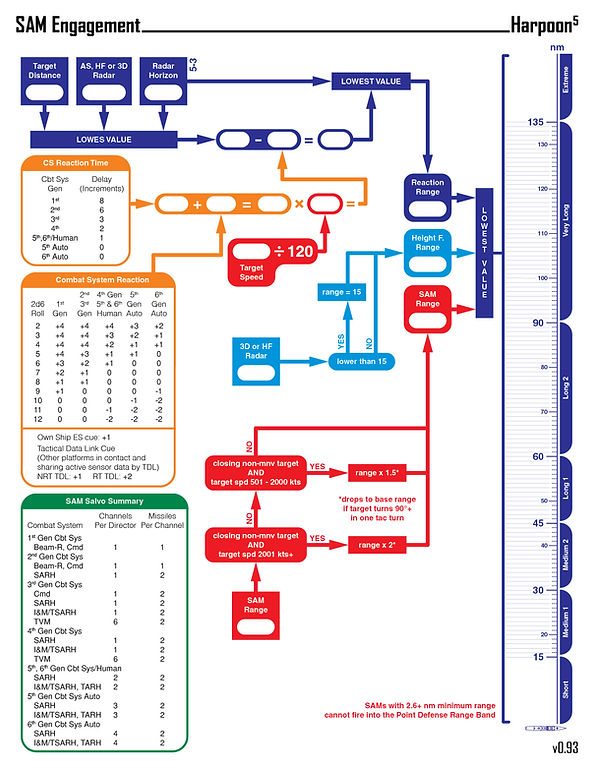
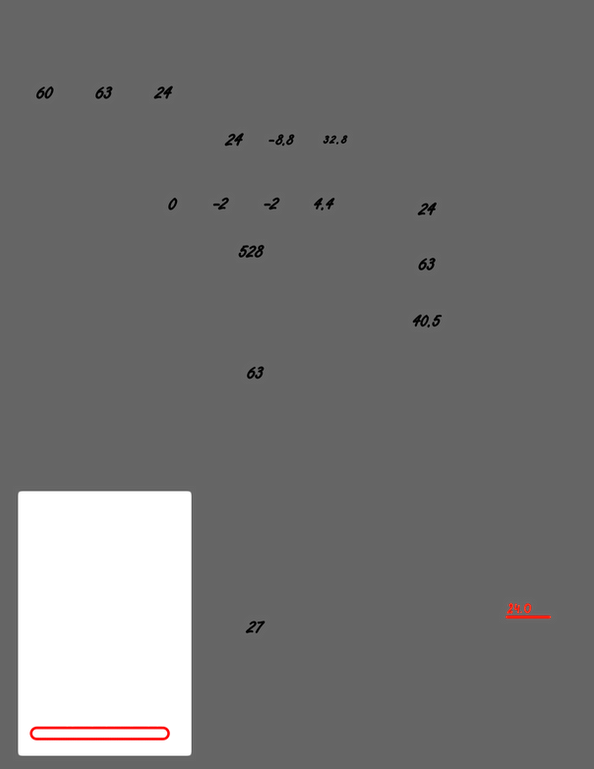
So having three directors, each able to guide missiles at up to four targets, might mean that Roosevelt can engage up to 12 targets in each engagement segment. Unfortunately it's not always the case.

Although search radars always have 360 degrees coverage and VLS can fire in any direction, weapon directors are almost always limited in arc they cover, and it's also the case for Burke's SPG-62s. The front director covers the Forward (F) 120 degree arc and two stern directors cover the Aft (A) 120 degree arc.
​
So 12 targets can be engaged if they are coming from the "broadside" , where all directors overlap. The worst scenario for Roosevelt are ASCMs coming in from the front 60 degree arc, rear arc is a little less worst off.
​
Since we assume worst case scenario for the Chinese attack, we will assume YJ-18A coming in from the "broadside" arc.
​
So 12 incoming YJ-18A can be engaged in Medium (M) band, another 12 in Short (S), yet another 12 in Short (S) again and finally 12 in Point (P) band. Each of these twelve attacks is declared, performed and evaluated before going for the next twelve. So if enough targets is destroyed in one band, there might be fewer attacks in the next band and some attacks may or may not be used to saturate remaining targets.
This large number of attacks create a bit of the problem for Burke if a lot of targets are coming in for her. If we assume infinite number of targets and two missile salvos against each of them, Roosevelt will run out of ESSMs by the first Short (S) band.

Fortunately for Roosevelt, Arleigh Burke IIA class ships posses also 58 SM2MR anti-air missiles. They are slightly less accurate than ESSM, but they have much longer range. This longer range doesn't help against sea-skimming targets though, because of the limiting factor of radar horizon. Still, they are very capable against ASCMs, the ESSMs are just a little better.
​
So, the final number of attacks against incoming YJ-18A, with maximum possible saturation will be:
​
M (Medium): 12 attacks x2 ESSM - left: 8 ESSM / 58 SM2MR
S (Short) 1st: 4 attacks x2 ESSM, 8 attacks x2 SM2MR - left: 0 ESSM / 42 SM2MR
S (Short) 2nd: 12 attacks x2 SM2MR - left: 0 ESSM / 18 SM2MR
P (Point): 9 attacks x2 SM2MR - left: 0 ESSM / 0 SM2MR
​
There are only nine attacks in Point (P) band, because at this point Roosevelt would run out of missiles. But as we will see soon, there would need to be a lot of ASCMs coming in to create such situation, much more than probably can be mustered at one point in time by Chinese.